Introduction
Euglena gracilis is a eukaryotic unicellular flagellate and belongs to the phylum Euglenozoa. It is found in freshwater habitats, quite often in eutrophic ponds and lakes (Tahedl and Häder, 2001; Azizullah et al., 2013; Borowitzka, 2018). The salient characteristic of E. gracilis that allows it to adapt to different environmental conditions is that its growth can be photoautotrophic (organisms that synthesize all necessary organic matter from inorganic substances using the energy from sun-light), heterotrophic (organisms that cannot produce their food, but instead avail nutrition from other sources of organic carbon, mainly plant or animal matter), or mixotrophic (organisms that follow a combination of both regimes) (Šantek et al., 2010; Buetow, 2011; Wang et al., 2018; Gissibl et al., 2019). E. gracilis can metabolize a wide range of alternative carbon sources such as glucose, glutamate, malate, pyruvate, lactate, and ethanol (Jasso-Chávez et al., 2005; Rodríguez-Zavala et al., 2010). Euglena species are extremely resistant to stressful conditions such as low pH (below 4), temperature changes, and high concentrations of metal ions (Wang et al., 2015; Gissibl et al., 2019). In addition, studies show that Euglena species can actively accumulate biomass in domestic wastewater with a high content of biogenic elements, namely nitrogen and phosphorus (Mahapatra et al., 2013; Kuroda et al., 2018), and reduce their concentration by 98% and 85% (Mahapatra et al., 2013), respectively, which makes them successful bioagents for wastewater remediation.
The removal of nitrogen and phosphorus is an important step in wastewater treatment because the presence of a large amount of nutrients with wastewater in water bodies results in unbalanced eutrophication, which in turn leads to dangerous consequences such as “bloom” of cyanobacteria, accumulation of toxic substances in the aquatic environment, violation of the oxygen regime, etc. (Ryding and Rast, 1989; Ansari et al., 2011; Li et al., 2012; Hwang, 2020; Lin et al., 2021; Preisner et al., 2021). This issue needs to be addressed not only for nature conservation and fishery purposes but also to overcome difficulties arising from drinking water supply and industrial water supply from eutrophic reservoirs. To prevent eutrophication and pollution of water bodies with phosphorus and nitrogen, a strict regulation of their concentrations in wastewater discharges has been established (Silva et al., 2015; European Commission, 2016; Mohsenpour et al., 2021). Taking into account the population equivalent (p.e.) defined by the EU legislation (Directive 91/271/EEC), wastewater discharges should meet the following requirements: for 10 to 100 thousand p.e. – total phosphorus and nitrogen content of up to 2 and 15 mg/l, respectively; for more than 100 thousand p.e. – total phosphorus and nitrogen content of up to 1 and 10 mg/l, respectively (Commission Directive 98/15/EC; Silva et al., 2015; Mohsenpour et al., 2021).
Microalgae are environmentally friendly and economical alternatives to traditional biological wastewater treatment processes (Abdel-Raouf et al., 2012; Molazadeh et al., 2019; Emparan et al., 2019; Pavliukh et al., 2020). Many researchers have reported high efficiency of using microalgae at various stages of wastewater treatment, both in the primary technology and at the final stages (Abdel-Raouf et al., 2012; Emparan et al., 2019; Mohsenpour et al., 2021; Geremia et al., 2021). The rationale for the use of mixotrophic microalgae in wastewater treatment is associated with the high lability of their metabolism and their ability to absorb inorganic and organic substances, thus decreasing their concentration in water (Kotoula et al., 2020; Geremia et al., 2021; Nezbrytska et al., 2021).
This research aimed to assess the ability of E. gracilis G.A. Klebs to reduce phosphorus and nitrogen concentrations in domestic wastewater to the level recommended by the EU legislation in a short time.
Materials and methods
Composition of wastewater
Synthetic domestic wastewater was used in the experiments. The chemical composition of synthetic wastewater was based on the formulation of a modified nutrient medium for the cultivation of euglenoid algae (Gromov, 1983): NaCl 0.1 g/l; MgSO4 × 7H2O 0.4 g/l; CaCl2 × 2H2O 0.05 g/l; glucose 5 g/l; α-glutamic acid 1 g/l; solution of microelements (g/l: H3BO3 2.86; MnCl2 × 4H2O 1.81; ZnSO4 × 7H2O 0.222; MoO3 0.018; and NH4VO3 0.023) 1 ml; and solution of iron EDTA [sodium iron (Fe3+) ethylenediaminetetraacetic acid (EDTA)] (g/l: FeSO4 × 7H2O 24.9 and EDTA 30.2) 1 ml. As the sources of phosphates and ammonium nitrogen, KH2PO4 and NH4Cl, respectively, were added to the medium to achieve the following concentrations of phosphorus and nitrogen:
Microalgae strain and culture conditions
Cultures of unicellular algae E. gracilis G.A. Klebs (strain HPDP-114) were obtained from the Collection of Microalgae Cultures of the Institute of Hydrobiology of the National Academy of Sciences of Ukraine (HPDP, Culture Collection of Algae in Hydrobiological Practice, Distribution and Perspective), Kyiv, Ukraine. Before using the cultures in the experiments, preliminary adaptation of the cultures to the studied compositions of wastewater was carried out (the adaptation period was 7 days). A stock culture of E. gracilis HPDP-114 was grown in 500-ml glass flasks containing 250 ml of modified Gromov’s medium (1983). After a 7-day culture period, a suspension of algae cells in the exponential growth phase was obtained, which was used as an inoculum. The microalgae were inoculated in 500-ml glass flasks, which contained synthetic wastewater. The ratio of inoculum to wastewater was 1 : 10. The initial concentration of microalgae biomass was 120 mg/l. Euglena gracilis was cultivated in wastewater at 27 ± 2°C under artificial lighting using fluorescent lamps Philips TL-D 18W 54-765 G13 daylight.
Analytical methods
Sampling for analysis was performed on 0, 1, 2, 3, and 4 days of culturing of the microalgae. Concentrations of ammonium nitrogen and phosphate in water were investigated following the protocol of Arsan et al. (2006).
The percentage of nutrients removed from wastewater by the microalgae was calculated using equation (1):
where C0 is the initial concentration of nutrients and Ct is the concentration of nutrients at time t.
Growth intensity of the culture under the studied conditions was evaluated by changes in dry weight (DW). The algal cell suspension was filtered through predried and weighed cellulose nitrate membrane filters (Sartorius, pore size 0.45 μm). The filters with the precipitated algae were dried to a constant weight in a thermostat at 105°C (Lim et al., 2010). Dry matter content was calculated using equation (2):
(2)
Biomass productivity was calculated using equation (3):
where N (mg/l day−1) is the concentration of biomass at the end of cultivation, N (mg/l day−1) is the concentration of biomass at the beginning of cultivation, and T is the duration of cultivation (4 days).
The chlorophyll a content and the sum of carotenoids were determined using the extract spectrophotometric method (SCOR-UNESCO, 1966). To determine the content of photosynthetic pigments from each flask in which algae were cultured, 10 ml of cell suspension was used. Sampling for analysis was performed on 0, 1, 2, 3, and 4 days of algae cultivation. Algae cells were separated from the culture medium by filtration using cellulose nitrate membrane filters (Sartorius, pore size 0.45 μm) under low vacuum conditions. To extract pigments, a filter with concentrated algae cells was placed in a porcelain mortar and ground with the addition of quartz sand and 90% acetone. Spectrophotometry of the extracts was carried out at the wavelengths of 664, 647, and 480 nm, which correspond to the maxima of light absorption in 90% acetone, respectively, for chlorophyll a, chlorophyll b, and carotenoids (SCOR-UNESCO, 1966). In addition, nonspecific absorption of light by the extracts was measured at the wavelength of 750 nm. The concentration of chlorophyll was calculated using the equation of Jeffrey and Humphrey (1975), and the sum of carotenoids was calculated using the equation of Parsons and Strickland (1963).
Statistical analysis
All indicators were determined in triplicate. The results were statistically analyzed using the program SPSS Statistics (version 17). Arithmetic means and standard deviations were calculated, and Student’s t-test was carried out. The difference was considered significant at P ≤ 0.05.
Results
Percentage of phosphorus and nitrogen removal from wastewater using E. gracilis
The most active removal of phosphates (
Table 1
Percentage of
Variant of experiment | Day of experiment | |||
---|---|---|---|---|
1 | 2 | 3 | 4 | |
I | 7 | 14 | 47 | 63 |
II | 6 | 16 | 28 | 43 |
III | 4 | 18 | 31 | 44 |
Fig. 1
Changes in phosphorus (A) and nitrogen (B) concentrations in Euglena gracilis culture media during the 4-day experiment: I – 4 mg
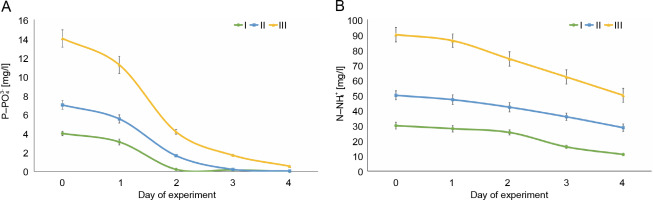
The percentage removal of ammonium nitrogen (
Changes in the intensity of E. gracilis growth
To assess the growth intensity of E. gracilis during its cultivation period in wastewater, changes in dry mass were studied (Fig. 2). On the first day of the experiment, the lowest rates of biomass accumulation were observed in all variants of wastewater, which is consistent with the lowest rates of removal of nutrients on the first day. Starting from the second day, biomass production increased due to the transition of the culture from the lag phase to the phase of intensive growth. As a consequence, the nutrient removal efficiency also significantly increased (see Table 1 and Table 2), which should be taken into account when using microalgae in wastewater treatment. At higher concentrations of nutrients, the microalgae were characterized by higher indicators of biomass accumulation. For the variant with 4 mg
Changes in the content of chlorophyll a and the sum of carotenoids in E. gracilis
The photosynthetic activity of E. gracilis was determined by the change in the content of photosynthetic pigments, chlorophyll a and sum of carotenoids (Fig. 3). On the first day of culture in wastewater, no significant increase in the content of photosynthetic pigments, as well as dry mass, was observed in any variant of the experiment. Starting from the second day, positive dynamics of the accumulation of chlorophyll a and carotenoids were observed for all studied compositions of wastewater. During the first 3 days, no statistically significant difference (P > 0.05) was observed between the values of the content of photosynthetic pigments. By the end of the experiment, when E. gracilis was cultivated on wastewater with 4 mg
Discussion
In this study, E. gracilis was characterized by its ability to remove ammonium nitrogen and phosphates from wastewater under the studied conditions. It removed inorganic phosphorus from the aquatic environment more efficiently than inorganic nitrogen, which could be attributable to their different initial concentrations in wastewater. In addition to NH4Cl, which was the source of the inorganic nitrogen, α-glutamic acid (C5H9NO4) was present in synthetic wastewater, which is a source of organic nitrogen. According to Buetow (2011), Wang et al. (2018), and Gissibl et al. (2019), E. gracilis is capable of mixotrophic growth and uses both inorganic and organic substances to maintain metabolism, which further explains the lower percentage of inorganic nitrogen removal from wastewater. The percentage of ammonia nitrogen removal by E. gracilis increased with an increase in the duration of exposition (up to 3 and 4 days), which, of course, is associated with a decrease in the concentration of organic nitrogen. Previous studies (Oswald and Gotaas, 1957; Abdel-Raouf et al., 2012; Emparan et al., 2019) have reported that photosynthesis plays a key role in the removal of inorganic nitrogen and phosphorus compounds from wastewater by microalgae. The results of the present study are consistent with this observation, as the content of photosynthetic pigments in E. gracilis increased with an increase in the period of exposition and the nutrient concentration in wastewater. According to Kitaya et al. (1998), the efficiency of photosynthesis in E. gracilis was about 60 times higher than in rice and 2 times higher than in algae of the genus Chlorella. Thus, due to this high photosynthetic activity, E. gracilis can increase the concentration of oxygen in wastewater, which has a positive effect on the oxidation and mineralization of organic impurities.
Analysis of the data obtained showed that E. gracilis is characterized by a higher efficiency of phosphorus removal from wastewater than some green microalgae from the genera Chlamydomonas and Chlorella (Kong et al., 2010; Bhatnagar et al., 2010; Ruiz-Marin et al., 2010). According to Kong et al. (2010), Chlamydomonas reinhardtii P.A. Dangeard removed 12.5 to 15.4% of
Despite these advantages, some practical problems may limit the use of E. gracilis in wastewater treatment. As reported in the literature, the major problems of using microalgae in tertiary wastewater treatment are biomass harvesting and utilization because secondary pollution of wastewater or environmental pollution can occur due to the decomposition of algal biomass (Pandian and Thomas, 2019; Molazadeh et al., 2019; Geremia et al., 2021).
According to previous studies (Pittman et al., 2011; Abdel-Raouf et al., 2012; Mahapatra et al., 2013; Eladel et al., 2019; Pavliukh et al., 2020; Geremia et al., 2021), the accumulated algal biomass in wastewater can serve as a raw material for the production of industrially valuable metabolites. It has been reported that E. gracilis accumulated a significant amount (up to 50–80% of DW) of paramylon, a type of β-1,3-glucan that is unique to Euglenozoa, in particular species of the genus Euglena (Suzuki et al., 2013; Grimm et al., 2015; Sun et al., 2018; Harada et al., 2020). In addition, E. gracilis has been investigated as a source of lipids (up to 20% of DW) for biofuel production and proteins (33–61% of DW) (Wang et al., 2015; Gissibl et al., 2019; Khatiwada et al., 2020). The fatty acid profile of E. gracilis is characterized by a high content (> 50% of total lipid) of polyunsaturated fatty acids, including α-linolenic, arachidonic, eicosapentaenoic, and docosahexaenoic acids (Korn, 1964; Wang et al., 2015). Thus, by appropriate treatment, the accumulated biomass of E. gracilis can be converted into fuel or additional raw materials to obtain other commercially valuable products.
For microalgae biomass harvesting from wastewater, filtration, centrifugation, flocculation, flotation and immobilization, as well as various combinations of these methods, are currently the most widely used methods (Larsdotter, 2006; Bhatt et al., 2014; Geremia et al., 2021). Some of these methods are labor and energy intensive (e.g., filtration and centrifugation) or accompanied by the addition of chemicals to wastewater (e.g., flocculation), and hence, their use is limited (Bhatt et al., 2014). Previous studies have reported that many species of algae (such as Botryococcus braunii Kützing, Chlorella vulgaris Beyerinck [Beijerinck], Chlorella ellipsoidea Gerneck, Neochloris oleoabundans S. Chantanachat and Bold, Scenedesmus obliquus (Turpin) Kützing, etc.) are characterized by their biosedimentation and bioflocculation abilities, which facilitate the collection of their biomass, but these abilities are less pronounced in flagellated microalgae (Stahl, 1880; Reynolds, 1984; Larsdotter, 2006; Salim et al., 2011; Moorthy et al., 2017; Patyna et al., 2018). To summarize, there is a need for further research aimed at developing effective methods for harvesting E. gracilis biomass.
Conclusions
The E. gracilis Klebs HPDP-114 strain can reduce the concentration of nutrients (nitrogen and phosphorus) in domestic wastewater that cause eutrophication of water bodies. In this study, 96–100% of phosphates (
The removal of E. gracilis biomass from wastewater is the primary problem that limits the use of this flagellated microalgae in wastewater treatment processes. Therefore, further research is needed to develop cost-effective and environmentally friendly methods for the harvesting and utilization of microalgae biomass.